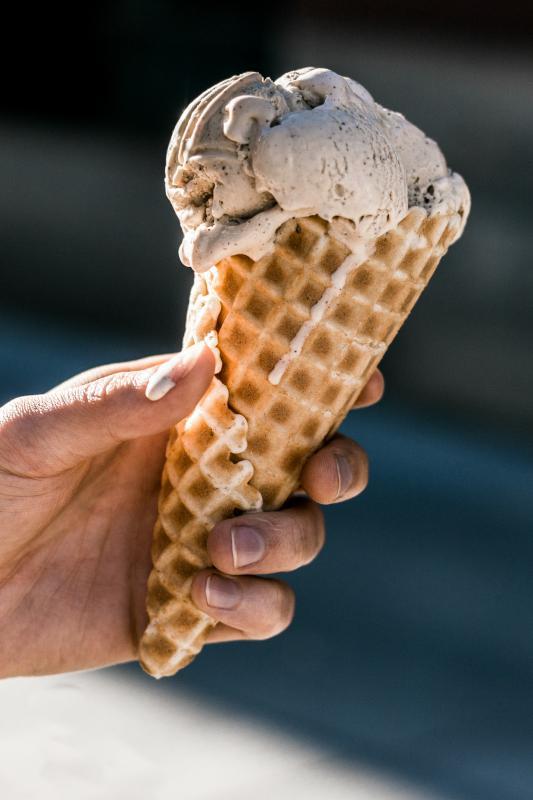
Details
Solution type
Topical area
Demand-side technologies for district heating and district cooling affect and are affected by changes in temperatures. There are mainly two questions in focus:
- What technologies can be used when building new buildings for low-temperature district heating or high-temperature district cooling?
- How can existing buildings be adapted or retrofitted for low-temperature district heating or high-temperature district cooling supply?
There is a difference in the preconditions regarding space heating and domestic hot water. Both production and distribution need to be looked at specifically. Connected to this is also the issue of reducing peak loads, and the possibility to connect the systems to a district heating grid. When working with temperatures below 45°C, there is also a challenge regarding legionella.
Low-temperature space heating technologies
Since space heating is a low-temperature demand – its purpose is to provide an indoor temperature of around 20°C – building or adapting space heating systems to suit low-temperature heat supply is a matter of design parameters. The three prevailing low-temperature space heating technologies are radiators, underfloor heating and forced-air heating. These technologies, and how they can be adapted to suit low-temperature district heating, are described in this section.
Radiators
A radiator is a very common technology for providing space heating and acts simply as a heat exchanger between the building's hydronic heating system and the room. Radiators can be designed for a range of temperatures, including levels that are suitable for low-temperature district heating supply, for example, 55°C/25°C supply/return. Lower temperatures mean increasing the size of the radiators (a larger surface area is needed to deliver the same amount of heat), but this is usually compensated for by lower heat demands in new buildings[1]. Radiators in existing buildings are usually designed for higher temperatures. The temperature level varies between and within countries due to different design criteria used historically. Often, radiators are designed to suit boilers that operate at high temperatures. For example, in Sweden, most systems were traditionally designed for 80/60°C (supply/return temperature), but since 1985 most systems are designed for 55/40-45°C[2]. There are basically three options available for reducing the supply temperature to existing buildings with radiators:
- Reduce the temperature as much as possible without any modifications. Due to the fact that many radiator systems are over-sized (for example because a larger size was installed to prevent draught under windows), and many houses have undergone some kind of refurbishment to reduce energy use (for example installing new windows), the temperature can often be reduced to a certain level without affecting the thermal comfort.
- Replace the radiators. By installing low-temperature radiators, the heat demand can be supplied with a lower temperature. This saves no energy but is usually cheaper and faster than energy refurbishments.
- Refurbish the house to save energy. By reducing the heat power needed to reach thermal comfort, existing radiators can be supplied with lower temperatures. If combined with replacing the radiators, the supply temperature can be reduced even further.
In a simulation of a typical Danish single-family house, it was found that the first option would enable a maximum temperature of 60°C for 92 % of the year[3]. Thus, by raising the supply temperature during about 700 hours per year, an existing DH network could be operated mostly as a low-temperature system without any modification of the space heating systems. This figure could be even lower in reality, since most existing buildings already have new windows. Replacing the radiators or refurbishing the house (in this case with low-energy windows and attic insulation) allowed for a maximum temperature of 59°C throughout the year. Doing both reduced it to 50°C. Low-temperature DH case studies using this technology: Aarhus, Lystrup (Denmark), Slough (UK), Aarhus, Tilst (Denmark), Västerås (Sweden), Stavanger, Østre Hageby (Norway)
Underfloor heating
Hydronic (waterborne) underfloor heating systems provide space heating by heating the floor, using pipes laid in the floor to circulate hot water. Because of the large surface area, heat can be delivered with low temperatures, only a few degrees above the indoor temperature. Also, for comfort reasons the floor should not be too much warmer than the indoor air. Underfloor heating is thus very well suited for low-temperature district heating supply. It can also be beneficial for reducing the return temperature. Underfloor heating reacts very slowly to variations in the demanded heat loads, which can lead to reduced comfort. On the other hand, this may also lead to a decrease of the demand-side peak power. Since it is usually costly to retrofit an existing building with this technology, underfloor heating is best suited for new buildings, but it can also be an option for extensive renovations. Underfloor heating may be used in combination with other solutions, for example to provide comfort heating in bathrooms even if the rest of the rooms are equipped with radiators. Low-temperature DH case studies using this technology: Albertslund (Denmark), Aarhus, Lystrup (Denmark), Høje Taastrup, Sønderby (Denmark), Västerås (Sweden), Stavanger, Østre Hageby (Norway), Kassel, Zum Feldlager (Germany)
Forced-air heating
Forced-air heating means space heating is supplied by warming the supply air in a building with forced ventilation, i.e. the heating system is combined with the ventilation system. For comfort reasons, and because warm air rises to the ceiling, the supply air must not be too warm. If the heat demand is large, this means that the air may need to be mixed with recirculated indoor air. In low-energy buildings, however, especially in passive houses where this is a common choice, forced-air heating may suffice without increased ventilation or supplementation with radiators or underfloor heating. Among its benefits are low installation costs (no extra system for heat distribution is needed) and rapid control. A drawback is that the temperature is often controlled centrally, with no means to control the heat supply for each room individually. Low-temperature DH case studies using this technology: Slough (UK), Västerås (Sweden), Okotoks (Canada)
Combination of waterborne and airborne systems
Waterborne systems have been used to a large extent in residential and non-residential buildings due to their high energy and comfort properties. These low-temperature heating systems have been applied to efficient buildings with high thermal insulation and airtightness. A problem that can arise is that the building might have too little fresh air infiltration, which can lead to health issues. To avoid such problem one can integrate waterborne and airborne systems[4].
Low-temperature domestic hot water technologies
Production and distribution of domestic hot water
Figure 1. Sketch of a typical domestic water installation. The incoming cold water is lead to the taps and to the house substation. In the case of hot-water circulation, the DHW is circulated back to the substation in order to maintain a high between tapings to reduce waiting time. Picture by SP.
We use domestic cold water for various purposes in our homes. The cold water comes into the building through a pipe, see Figure 1. Part of the incoming cold water is used as cold water and the other part (typically about 30-40%) is heated to domestic hot water (DHW). The DHW is heated in the building by transferring heat from e.g. the district heating network. In a substation, the water from the DH is used to heat the domestic cold water by a heat exchanger. The DH water and the domestic water are therefore separated, and only heat is exchanged. In this process, some temperature degrees are always lost, which results in the DHW being about 5˚C lower than the DH distribution temperature. The DHW can be heated instantaneously or stored in a hot water tank in the substation. The DHW is then distributed to the different taps in a building directly from the substation or through a hot-water circulation circuit.
Instantaneous heating
The simplest way to produce domestic hot water with district heating is through instantaneous heating using a heat exchanger. In this way, DHW is only produced when needed and no storage is needed. However, the substation must be able to supply a sufficiently high heat capacity and handle quick variations in DHW demand. In a low-temperature system, the difference between the incoming district heating supply temperature and the required DHW temperature will usually be small, which means that the heat exchanger needs to be bigger than in standard systems. In Danish low-temperature systems, heat exchangers with three to five times larger plate areas have been used[1]. Euroheat & Power recommends instantaneous DHW heating in its guidelines for DH substations[5]. Low-temperature DH case studies using this technology: Aarhus, Lystrup (Denmark), Slough (UK), Høje Taastrup, Sønderby (Denmark), Västerås (Sweden), Stavanger, Østre Hageby (Norway)
DHW storage
By storing DHW in a storage tank, variations in the demand can be evened out and peak demands will be lower. However, storing hot water means an increased risk of bacterial growth (Legionella), and the temperature needs to be higher than when heating DHW instantaneously. Low-temperature DH case studies using this technology: Kortrijk, Venning (Belgium)
Hot-water circulation circuit
To guarantee that the DHW reaches the tap within a reasonable time in a large building, a hot-water circulation circuit can be used. A pump circulates the DHW from the most distant tap back to the heat source. In that way the water is kept warm even when no DHW is used. Due to the risk of legionella, the temperature of the circulating water should never be lower than 50°C. This, combined with the relatively high heat losses from the hot-water circulation, implies that this technology is not very appropriate for low-temperature systems.
DH storage
Another option, as opposed to DHW storage, is to store DH water. By buffering DH water, variations in the heat demand can be evened out while still preparing DHW instantaneously in a heat exchanger. This means peak loads are reduced, which affects the dimensioning of DH pipes – smaller flows mean smaller pipes can be used, which means reduced distribution losses, see Reduction of peak loads. However, there will be heat loss from the DH storage tank, which may counteract the gain on the distribution side. The DH storage is, in contrast to DHW storage, a case where the stored water is used only as an energy carrier (it is not used as DHW), which means that the temperature in the DH storage has no limitations imposed from the risk of legionella. This design has been investigated both in practice (Aarhus, Lystrup (Denmark)) and in theory[6], showing promising results. It has also been shown to enable lower heat losses in the DH system, thanks to lower water flows[7]. Compared to DHW storage, the supply temperature can be lower, but it is also a more expensive solution in terms of investment costs. Low-temperature DH case studies using this technology: Aarhus, Lystrup (Denmark)
Booster heat pumps
An option that allows supply temperatures even lower than 50-55°C is to use another heat source to boost the DHW temperature. One such option is to use a small heat pump – a “booster heat pump” or “micro heat pump”. By combining it with a storage tank, the heat power needed for raising the temperature from the DH temperature to the required DHW temperature can be small, and the heat pump can operate efficiently. The heat pump can either heat the DHW or the DH water, and the storage tank can be of either the DHW or the DH type.
In both cases, the storage also has the added benefit of evening out the heat load and reducing peak loads. However, since electricity is used, the benefits of lowering the temperature below ordinary low-temperature DH levels must be bigger than the drawback of using high-quality energy for heating purposes. There are various technical solutions to this approach. One is to let the heat pump heat the DHW water. Another option is to boost the district heating water before heating the DHW water in a standard heat exchanger. Concepts for a supply temperature of 40 °C have been developed in Denmark, using efficient “micro heat pumps” (COP 5.3)[8].
Booster heat pumps are also an option if a low-temperature system is used in areas where just a few customers have higher temperature demands (for example due to older space heating systems). Instead of operating the entire system at a higher temperature level to meet the demand of just a few buildings, these specific buildings could be equipped with heat pumps. Low-temperature DH case studies using this technology: Albertslund (Denmark)
Reduction of peak loads
The decrease of peak loads is a very important issue for the future development of the district heating sector and especially for LTDH[9]. The demand side sets the limit on the maximum amount of heat being produced and distributed in the network. Thus, by decreasing the maximum need of heat at a particular time – the peak load – one can decrease the scale of the production and the distribution – the peak power – as they need to be able to deliver heat “in the worst case scenario”.
In this section, the aspects of peak loads that are related to LTDH are discussed. Further information about peak loads in general, methods peak shaving etc. is available here: Decreasing peak loads/Demand management (and in Celsius deliverable D5.2). The decrease of the peak loads in the DH network comes from the decrease of the peak loads of the demand side SH (space heating) and DHW. Thus, a decrease of the demand side peak loads and consequently in the demand side peak power results in a decrease of the supply and distribution peak loads and peak power dimensioning[10].
The benefits of reducing the peak loads in a DH network include possible reduction of diameter of pipes in as they are dimensioned for the peak loads. This leads to lower heat losses and reduced investment costs. For the production side, the reduction of peak loads leads to optimized/reduced sizing of peak power production of heating plants and reduced need for primary heat storage. It also implies that the heating plant can be operated for continuous power production instead of intermittent production.
The reduction of the demand side peak power can be accomplished by decreasing the energy consumption for SH and optimizing the SH and DHW systems. The peak power demand (the dimensioning of the branches to a given building) is given by the DHW in the case of instantaneous heat exchangers. In that case, optimization of the SH does not have an effect. In the case of a heat exchanger with DH storage, the peak power is given by the combination of the peak power from DH and DHW.
Reduction of peak loads for space heating
Thermal capacity of building
The peak power can be reduced by decreasing the energy demand for SH of the building through refurbishment of the building envelope, the infiltration and ventilation system and of the windows. These kinds of measures decrease the general heat demand, and thus also the peak loads, even if the relative fluctuations in demand are not necessarily affected. The peak power can also be reduced by using the thermal mass of the building to store heat, thus counteracting variations in the demand. If the load is well managed, indoor temperature variations will not be higher than normal. This is further described in the article Decreasing peak loads/Demand management (or Celsius Deliverable D5.2 Strategies to improve energy efficiency for end users and for peak shaving) and in Thermal energy storage and related articles (or Celsius Deliverable D5.6 'Concepts for storage and load control').
Heating systems
The use of low temperature heating can decrease peak power[9]. The use of underfloor heating with constant flow can give up to 40% lower peak power compared to on/off-control, while the use of low temperature radiators provides little possibility to decrease peak power.
Reduction of peak load for DHW
A reduction of the peak power load for DHW can be achieved by using DH storage. The peak power is then given by the charging flow rate of the buffer tank. There is a trade-off between the size of the buffer tank and the charging flow. On the one hand, a larger tank gives a lower charging flow rate, but on the other, a large tank has larger heat losses, needs a large space in the building and is more costly. As an intermediate and more flexible solution there is a possibility of having a smaller tank with about 20-60 litres[9].
Connecting space heating and DHW systems to DH
Substations
Figure 2. Sketch for traditional SH and DHW systems in a building. The DHW and the SH are produced centrally in the building and the hot-water circulation is used to guarantee that the DHW is supplied within a reasonable time for the taps far away from the house substation. Picture by SP
A district heating substation (a.k.a. heat interface unit) is the interface between the district heating system and the space heating and DHW systems of a building. The substation delivers heat to the building systems, thus replacing the boiler, heat pump or other equipment used in buildings without district heating.
A substation can be designed in various ways depending on the specifications of the building systems, the DH temperatures etc., but it typically includes heat exchangers, valves, control equipment, piping, filters and heat meters. It can also include pumps and a storage tank.
In the following, the focus is on temperature aspects for substations, providing information about aspects that are specific to low-temperature district heating and also about important aspects for achieving a low return temperature.
A general description of substations is available in Celsius Deliverable D2.1 on end users aspects. For more in-depth technical information on the design and choice of substations, Euroheat & Power’s guidelines for district heating substations[5] provide useful information. Also, a more recent document has been developed in CEN European committee for standardization, a so-called workshop agreement, CWA 16975 Eco-efficient Substations for District Heating, potentially to be transformed into a European standard. This is partially based on the Euroheat Guidelines, but has been further developed and, in addition to technical requirements, emphasises eco-efficiency and cost-effective production, aiming at more standardised and higher-quality substations[11]. The document is available through national standardisation bodies. A certification procedure based on the document is managed by Euroheat&Power.
In large building systems such as multi-family buildings with centralized DHW preparation, a circulation circuit is often used in order to enable the fast supply of domestic hot water, see Figure 2. In some places, this is also used in single-family houses. It is important to adjust the circulation system for correct flow rate and high enough temperature in all of the systems in order to avoid the growth of legionella bacteria.
Flat substations
Figure 3. Sketch for DHW and SH system in a building with flat substations. Only one tap and one radiator per flat are shown; in a real building the system is obviously more branched. The heat exchange to the local DHW and SH systems occur in every flat and no hot-water circulation is needed. Picture by SP.
Flat substations, or individual substations, as opposed to one central substation, can be used to enable low-temperature DH in multi-family buildings, see Figure 3. There are several reasons for using individual substations:
- By preparing DHW close to the tap, no DHW circulation is needed and the pipe volume with heated water is small, allowing for lower DHW temperature and thus lower DH temperature.
- Each flat is only supplied with two pipes with hot water (DH supply and return), as opposed to four (space heating supply and return, DHW and DHW circulation), significantly reducing heat losses[12]and piping costs.
- Smaller substations can be standardized and produced more efficiently, leading to lower manufacturing costs.
The disadvantage of the flat substation is that the installation costs are quite high[13] as the flat substations have to be installed in every flat. A complete economic evaluation is difficult to perform as the benefits from a decrease in the distribution temperatures are difficult to estimate and also need to include the lifetime of the installation. A flat substation can either be directly or indirectly connected to the DH system.
With indirect connection, district heating is first connected by a heat exchanger unit in the basement of a multi-family building to a secondary circuit in the building. Then DHW is connected individually in each flat with a heat exchanger/DHW storage to this secondary circuit. This is sometimes used when individual DHW production is desired at the same time as keeping the DH medium out of the flats. This solution implies a thermodynamic disadvantage - with two heat exchangers the temperature drops are higher, thus requiring a higher DH supply temperature to support the DHW and also resulting in a higher return temperature.
Indirect or direct connection of space heating
The space heating system of a building can be either directly or indirectly connected to the district heating system. A direct connection means the district heating water is used directly in the building's heating system. This works if the network pressure is not too high. The advantage is that there is no temperature drop between the district heating and space heating systems, thus allowing for both slightly lower supply temperatures and slightly lower return temperatures. In networks with higher pressures, an indirect connection is used, meaning that the systems are hydraulically separated with a heat exchanger.
This way, the pressure of the district heating network is independent of building installations, which is important in some cases, for example in hilly locations. The systems are clearly separated, which has a number of technical advantages (oxygen dissolved in the water increasing the risk of corrosion, risk of pressure surges, consequences in the event of a leak and increased risk for noise) (Frederiksen & Werner, 2013). However, with a heat exchanger, the district heating supply temperature needs to be a little higher, and the substation will be more expensive.
Direct and indirect connections are both used in traditional, standard-temperature district heating systems. Direct connection is common in, for example, Denmark and Germany, whereas indirect connection is common in, for example, Finland and Sweden[14]. Most of the low-temperature case studies described in this report use space heating directly connected to the district heating network.
However, these are small networks with low temperature and low pressure, which decreases the risk of leakage in comparison to larger systems. Some of them are also secondarily connected to a larger network with higher temperatures and pressures, why they in practice could be comparable to large space heating systems shared by several buildings, although with DHW connected in an additional step. The Danish Guidelines for low-temperature DH[1]prescribes that direct connection can be used for systems with design pressure below PN10 (i.e. max 10 bar).
Low-temperature DH case studies using direct connection: Albertslund (Denmark), Aarhus, Lystrup (Denmark), Slough (UK), Linköping (Sweden), Västerås (Sweden),
Low-temperature DH case studies using indirect connection: Høje Taastrup (Denmark), Linköping (Sweden), Kortrijk, Venning (Belgium)
Connection schemes
Figure 4. DH substation with a 2-step connection of DHW and space heating.
Figure 5. Simplified schemes of parallel connection of domestic hot water and space heating.
There are several options for connecting indirect space heating systems and hot water systems to district heating. The two most common options are parallel connection and 2-step connection, which are shown in Figures 4 and 5.
The Euroheat & Power Guidelines[5] recommends the parallel connection of space heating and DHW when the return temperature from the space heating system is not high. On the other hand, for high space heating return temperature (i.e. not low-temperature systems), a 2-step connection (pre-heating DHW by DH return from space heating heat exchanger) is recommended, since in some cases it decreases the DH return temperature, but the installation cost is a little higher.
Most of the low-temperature case studies described in this report use space heating directly connected to a small low-temperature district heating network. In these cases, DHW is connected via a heat exchanger to this circuit (sometimes with a storage tank on the DH side). This is also what is recommended in the low-temperature guidelines from Denmark[1]. For indirect space heating connections, these guidelines recommend the parallel connection of the two systems.
Heat exchanger temperature performance
When using indirect heating connection/instantaneous DHW, it is important to use high-performance heat exchangers to minimize the temperature drop from the district heating to the building systems and back to the district heating return pipe and also to avoid high pressure drops. This is even more essential in low-temperature systems than in systems with higher temperatures. Brazed plate heat exchangers are the most commonly used types. In some cases, especially in areas with water containing high levels of lime, gasketed plate heat exchangers are used in order to facilitate cleaning. However, high turbulence usually provides a self-cleaning effect with compact brazed plate heat exchangers. Tube heat exchangers are seldom used nowadays, as they are not as efficient and have a higher risk of breaking.
The CEN guidelines currently under development[11] will include requirements on heat exchanger sizing, stated as the maximum permitted difference between return temperatures on the primary and the secondary sides respectively, depending on district heating supply temperature and building system temperatures, and maximum permitted pressure loss. The sizing of control valves also affects the efficiency. It is not unusual that valves are oversized, leading to a risk of unstable and overly high flow rates, thus increasing the DH return temperature. This can be avoided by selecting valves according to heat loads and available primary side differential pressure.
By-pass
For instantaneous hot water preparation without a circulation system, a by-pass of district heating water is often used (within the substation or outside of it) in order to be able to supply hot water without having to wait for the district heating service pipes to heat up in seasons when no space heating is needed. However, it is essential to ascertain that there is no by-pass flow when not required and that the flow is not higher than necessary since it increases the return temperature.
Legionella in DHW
Temperature requirements
Safe temperatures of domestic hot water are quite narrowly defined by two different health risks: the risk of scalding with overly high temperatures and the risk of bacterial growth in water with overly low temperatures. The latter risk mainly concerns legionella, which are bacteria that can grow in the biofilm inside the pipes and can cause pneumonia when inhaled through aerosols (small droplets). As legionella grows in temperatures between 25°C and 45°C, minimum DHW temperature levels of 50-65°C are usually required. Table 1 shows the required temperature in a few countries. In its guidelines for DH substations[5], Euroheat & Power recommends that there should not be any connection of equipment to the system that could force the temperature below 50°C in any part of the system.
Consequently, the outgoing water temperature from the heat exchanger/storage tank should never be below 50°C in single-family houses and 55°C in multi-family houses. Taking distribution losses and temperature drop in the heat exchanger into account, this results in a minimum DH supply temperature of about 55°C, which is also the level used in several existing low-temperature DH systems. To reach the requirement in multi-family buildings, the temperature may have to be slightly higher, or flat substations may be used.
Country | DHW temperature at the tap (°C) | DHW production temperature |
---|---|---|
Belgium | ≥55 | ≥60 |
Denmark | ≥50* | ≥60 |
Finland | ≥55 | ≥60-65 |
France | ≤50 | ≥60 |
Germany (large systems) | ≥45 | ≥60 |
Italy | 45-48 | ≥60 |
The Netherlands | - | ≥60 |
Spain | ≥50 | ≥55 |
Sweden | ≥50 | ≥60 (in tank) |
United Kingdom** | ≥50 (45) | ≥60 (50) |
Table 1. DHW temperature requirements in some European countries. * The requirement of the DHW is 50 °C but 45 °C at maximum flow. ** There is no regulatory requirement to achieve a specific temperature. The figures cited are 'custom and practice' for commercial systems with hot water recirculation. (Real world domestic systems are more like 45C and 50C)' [16]
There are various ways of controlling legionella in the DHW system such as disinfection methods which kill or remove the bacteria and/or the biofilm[15], pipes with antibacterial properties that inhibit the establishment of the biofilm[17] and the small volume approach which minimizes the time the bacteria can grow (discussed below). The disinfection methods are currently mostly used in larger buildings such as hospitals, nursing homes or public baths. Pipes with antibacterial properties are still in the development phase.
Small DHW volume approach
The main barrier to reducing the DHW temperature below 50°C is the risk of legionella, as mentioned above. Apart from nutrients and favourable temperatures, legionella bacteria need time to grow. In a building with hot-water circulation, the water is constantly circulating, which means that it is very important that the temperature is kept sufficiently high, as otherwise the legionella will grow in the biofilm. In order to minimize the time the legionella is in the range of temperatures in which it grows, it is important to have DHW only when needed and minimize its volume[18], i.e. the volume from the heat exchanger and the tap. For single-family houses, this can be achieved by limiting the volume from the house substation and for a multi-family house this can, in practice, be achieved by using flat substations.
This approach has been used in some low-temperature systems in Denmark, where the DHW temperature has been set to approximately 45°C and its volume limited to 3 litres (Ottoson, 2013[3]; Christiansen, 2012; Rosa, 2012). Analyses from case in Lystrup have shown that DHW can be prepared at 46°C from a DH supply temperature of 50°C in these systems, with acceptable waiting times[19]. The Danish initiative is based upon an earlier German rule[20], which did not put any explicit limits on the DHW temperature for “small” systems. A system is considered ”small” if the hot water tank has a total volume of less than 400 litres and if the total volume between the heat source and the furthermost tap is less than 3 litres. In a later version of the rule[21], the temperature restriction was included for small systems, and now the lowest temperature is set to 50°C[22]. However, the efficiency of controlling the levels of legionella by limiting the volume of the DHW has not been scientifically verified. Low-temperature DH case studies using this technology: Aarhus, Lystrup (Denmark), Høje Taastrup, Sønderby (Denmark), Stavanger, Østre Hageby (Norway)
Other DH applications
District heating can also be used for other purposes than space heating and DHW, though these two are the most common ones. One relatively common type of application in many DH systems is industrial use of heat. This is usually very specific for the industries in question, and it is difficult to talk generically about their temperature requirements and the implications of lowered supply temperatures. However, if there are industrial customers in a DH system, their needs and requirements must be reviewed when investigating the possibility of using lower temperatures. There are also other possible applications of DH that should be considered, both applications that have been used in DH systems for a long time and new types of applications. These include:
- White goods (white goods technical article and Gothenburg white goods demonstrator).
- Ships (c.f.Gothenburg demonstrator and analysis in Celsius Deliverable D5.2).
- Heating of greenhouses for food production, green gardens, visitor centers etc.
- Ground heating to melt ice and snow in towns and bicycle lanes.
- Other types of public space heating, for example bus shelters.
- Heat driven cooling/absorption chillers.
All existing and possible future applications of DH should be considered when lowered temperatures are investigated. Some applications, e.g. ground heating, may be supplied with the return water, thus contributing to reducing the return temperature. Finding other DH and especially low-temperature applications is currently a topic that attracts a lot of interest, as it leads to increased competiveness of the DH companies. It is also in line with increasing energy efficiency, as a replacement of electricity by heat leads to a decreased use of primary energy.
References:
1. Olsen, P. K., Holm Christiansen, C., Hofmeister, M., Svendsen, S., Thorsen, J. E., Gudmundsson, O., & Brand, M. (2014). International guidelines for lowtemperature district heating.
2. Warfwinge, C., & Dahlblom, M. (2012). Projektering av VVS-installationer. Lund: Studentlitteratur.
3. Brand, M. (2014). Heating and Domestic Hot Water Systems in Buildings Supplied by Low-Temperature District Heating Heating and Domestic Hot Water Systems in Buildings Supplied by Low-Temperature District Heating. Technical University of Denmark.
4. Wu, X., Fang, L., Olsen, B. W., & Zhao, J. (2014). Proceedings of the 8th International Symposium on Heating, Ventilation and Air Conditioning, 261. doi:10.1007/978-3-642-39584-0
5. Euroheat & Power. (2008). Guidelines for District Heating Substations. Retrieved from http://www.euroheat.org/Admin/Public/DWSDownload.aspx?File=/Files/Filer/EHP_Guidelines_District_Heating_Substations.pdf
6. Paulsen, O., Fan, J., Furbo, S., & Thorsen, J. E. (2008). Consumer Unit for Low Energy District Heating Net. In The 11th International Symposium on District Heating and Cooling.
7. Olsen, P. K., Lambertsen, H., Bøhm, B., Christiansen, C. H., Svendsen, S., Larsen, C. T., & Worm, J. (2008). A New Low-Temperature District Heating System for Low-Energy Buildings. Proceedings of the 11th International Symposium on District Heating and Cooling.
8. Zvingilaite, E., Ommen, T., Elmegaard, B., & Franck, M. L. (2012). Low temperature district heating consumer unit with micro heat pump for domestic hot water preparation. In Proceedings of DHC13, the 13th International Symposium on District Heating and Cooling (pp. 136–143).
9. EUDP. (2011a). CO2-reductions in low-energy buildings and communities by implementation of low-temperature district heating systems. Demonstration cases in EnergyFlexHouse and Boligforeningen Ringgården: Miscellaneous investigations. Energistyrelsen.
10.EUDP. (2011b). Demonstration af lavenergifjernvarme til lavenergibyggeri i boligforeningen Ringgårdens afd. 34 i Lystrup Energistyrelsen - EUDP 2008-II, 250.
11. CEN. (2015). CWA 16975 Eco-efficient Substations for District Heating
12. Park, B., Kim, Y., Jung, I., Lee, J. Y., & Im, Y. (2012). Assessment of heat loss reduction in two pipeline system with individual household substation. In Proceedings of DHC13, the 13th International Symposium on District Heating and Cooling (pp. 121–127).
13.Yang, X., Li, H., Fog, J. M., & Svendsen, S. (2014). ANALYSIS AND RESEARCH ON PROMISING SOLUTIONS OF LOW TEMPERATURE DISTRICT HEATING WITHOUT RISK OF LEGIONELLA. In 14th International Symposium on District Heating and Cooling.
14. Frederiksen, S., & Werner, S. (2013). District heating and cooling. Lund: Studentlitteratur.
15. Yang, X., Li, H., & Svendsen, S. (n.d.-b). Review of Various Solutions for avoiding critical levels of Legionella Bacteria in Domestic Hot Water System. DTU Civil Engineering.
16. Measurement of domestic hot water consumption in dwellings, Energy Saving Trust on behalf of DEFRA https://www.gov.uk/government/publications/measurement-ofdomestic-hot-water-consumption-in-dwellings
17. Puig von Friesen, M., Arinder, P., Lundin Johnson, M., Yarahmadi, N., Kjellin, M., Kurkinen, E.-L., & Thörnblom, K. (2014). Säkerställa vattenkvalitet vid 45 ˚ C med avseende på legionella - En förstudie för innovationsupphandling. SP Technical Research Institute of Sweden.
18. Swedish Standards Institute. (2012). Vattenförsörjning – Rekommendationer för att förebygga legionellatillväxt i tappvatteninstallationer i byggnader. Teknisk rapport SIS-CEN/TR 16355:2012.
19. Brand, M., Thorsen, J. E., & Svendsen, S. (2012). Numerical modelling and experimental measurements for a low-temperature district heatingsubstation for instantaneous preparation of DHW with respect to service pipes. Energy, 41(1), 392–400. doi:10.1016/j.energy.2012.02.061
20. DVGW. (1993). W 551: Technische Regel, Arbeitsblatt. DVGW, Deutscher Verein des Gas- und Wasserfaches e.V.
21. DVGW. (2014). Legionellen. DVGW, Deutscher Verein des Gas- und Wasserfaches e.V. Retrieved November 26, 2014, from http://www.dvgw.de/wasser/trinkwasser-und-gesundheit/legionellen/.
22. DVGW. (2004). Code of Practive W 551: Drinking water heating and drinking water piping systems; technical measures to reduce Legionella growth; design, construction, operation and rehabilitation of drinking wa- ter installations. Bonn: DVGW German Technical and Scientific Association for Gas and Water.
External links:
District heating substations:
-
- Euroheat&Power Certification of district heating substations
- Regulation for Swedish district heating substations from the Swedish district heating association
- Fairheat British Substation requirements and testing
- Testing of substations at RISE
Replicability |
Low |
Medium |
High |
---|---|---|---|
Authorizative easiness | x | ||
Adaptability to different climate conditions | x | ||
Technology easy-to-implement (No needs of specific technical requirements) | x | ||
Easy-to-implement (No needs of specific technical requirements) | x | ||
Easy-to-operate (No needs of specific technical requirements) | x | ||
Opportunity of integrating waste energy sources | x | ||
CAPEX needed for the deployment of the solution | x |
Related Celsius content:
Strategies and concepts for LTDH and HTDC
Distribution Technologies for LTDH/HTDCHigh Temperature District Cooling technology
Case studies of Low Temperature District Heating systems
Webinar: Low temperature district heating